Currently a member of Gazzola Lab, I am deeply passionate about exploring and understanding, using computational and theoretical tools, the physics involving flow–structure interaction (FSI), multiphase phenomena, interplay between elasticity and fluid dynamics of FSI systems and continuum viscoelasticity. Below, you can find brief descriptions of the research domains I’m involved in:
Soft/rigid body flow-structure interaction
Accurate and versatile solvers are the key to shed light on and dissect underlying physics in FSI systems presenting body elasticity/compliance, with potential applications in medicine, soft robotics, flow control and inertial microfluidics. With that in mind, my recent venture at Gazzola Lab with the help of my colleague Tejaswin Parthasarathy has led to the development of a robust, flexible numerical algorithm for solving incompressible flow–structure interaction problems for mixed rigid/soft body representations, within a consistent framework based on the remeshed vortex method. The preprint detailing our study with the algorithm, benchmarks and illustrations can be found here here with one of the illustration cases shown below: a rigid cylinder falling on an elastic trampoline, with both bodies submerged in a viscous fluid.
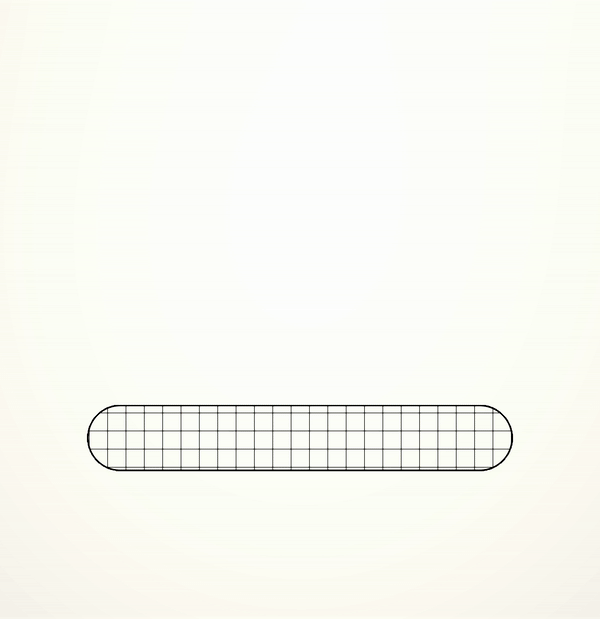
Viscous streaming
Viscous streaming refers to the time-averaged steady flow that arises when an immersed body of characteristic length scale D undergoes small-amplitude oscillations (compared to D) in a viscous fluid. With the broad range of applications of viscous streaming in microfluidic flow manipulation, particle trapping, scalar transport and passive swimming, our understanding of how streaming flow features and topology are affected by multiple body length scales remains largely incomplete. In my Master’s thesis work, we attempted to elucidate the mechanisms at play, when streaming flow topology is manipulated via regulated variations of shape geometry, thus providing a rational design approach for microfluidic applications. Our work, which can be found here has been published recently in Journal of Fluid Mechanics, with a glimpse of the effects of geometric variation on streaming flows shown below:
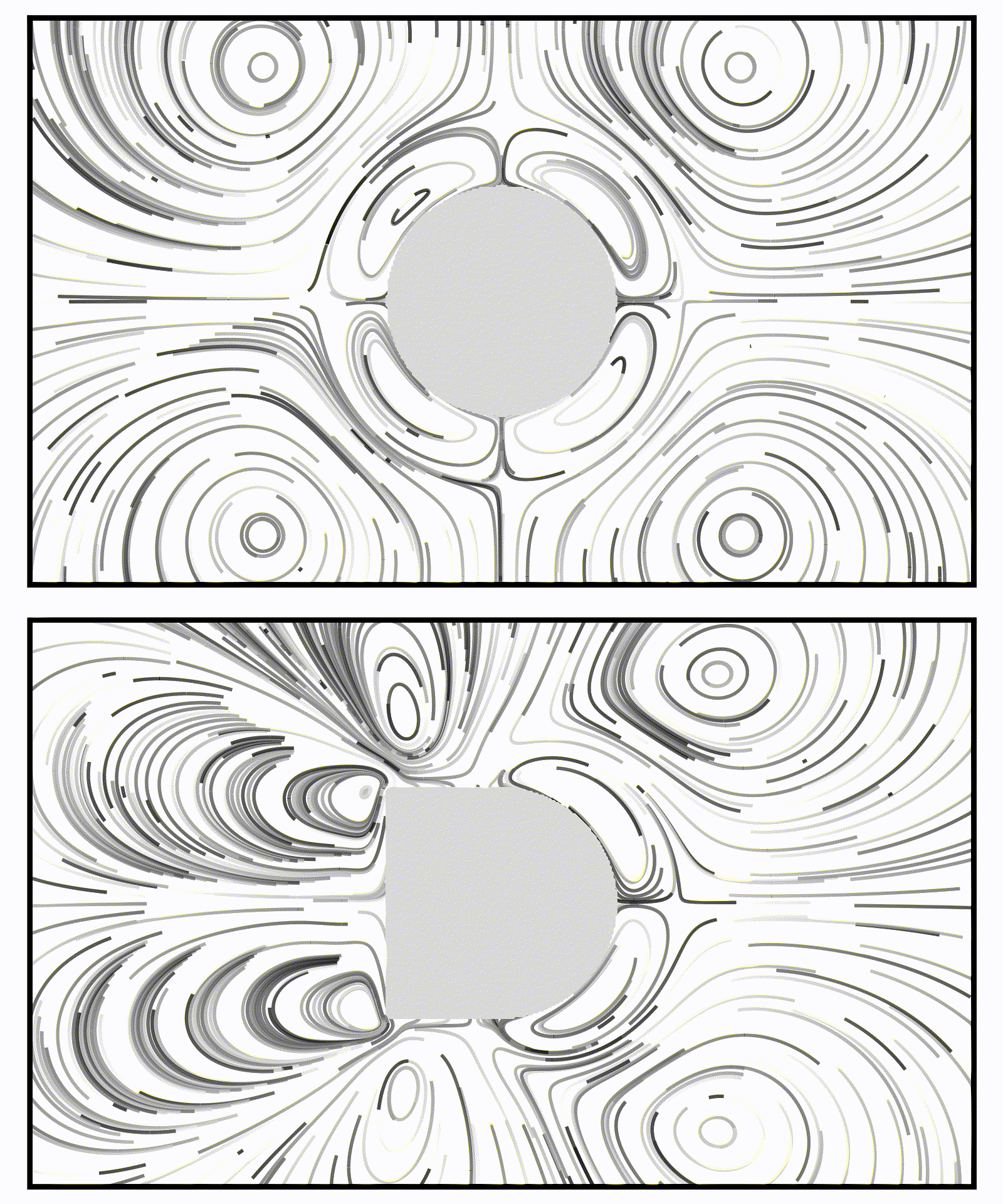
Dynamics of a leaf upon raindrop impact
Dynamics of drop impact on soft surfaces has drawn a lot of attention for its applications and is motivated by natural examples like raindrop impact on a leaf. Previous studies have focused on categorizing the bending motion observed, using cantilever beam theory, but the complex dynamic response shown by a leaf involving other degrees of motions like torsion about the petiole, remains yet to be understood. In a recent study, that can be found here, published in Bioinspiration & Biomimetics, we decompose the leaf’s motion as a superposition of multiple modes modeled as damped harmonic oscillators. Our results, that are in good agreement with experimental results, shed light on the design of potential raindrop energy harvesting devices mimicking a leaf’s structure. A video capturing the dynamics of a leaf’s response upon raindrop impact is shown below:
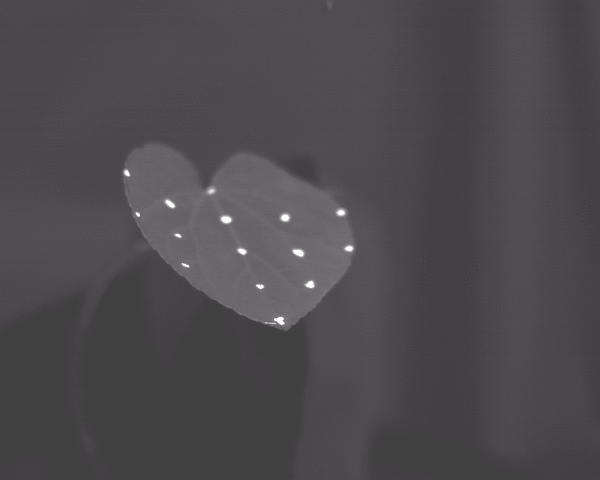
Emergent mechanics of a “bird nest” system
Systems of randomly packed, macroscopic elements, from jammed spherical grains to tangled long filaments, represent a broad class of disordered meta-materials with a wide range of applications and manifestations in nature. A bird nest presents itself at an interface between hard round grains described by granular physics to long soft filaments, the center of textile material science. In a recent Research Highlight published in Journal of Applied Physics (found here), while reviewing packing statistics, mechanical response characterization, and consideration of boundary effects, we present a perspective that attempts to establish a link between the bulk and local behavior of a pile of sand and a wad of cotton, demonstrating the nest’s relationship with each. Our research, in collaboration with Prof King, has been covered in New York Times (article link), with a characteristic compression cycle response of the computational bird nest systems shown below:
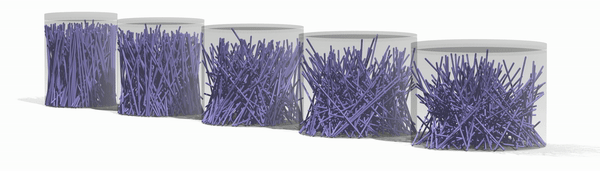
Natural convection characterization over a heated cylinder
Natural convection is widely used as a conventional method for cooling in various applications such as shell tube heat exchangers, electronic cooling, heat transfer through submerged pipelines, steam pipes in power plants and cooler pipes in nuclear reactors, due to its high reliability and low costs in design and maintenance. In my work with Prof K. C. Lin, we proposed a 3D-CFD model to predict the existing experimental data and heat transfer characterization for a natural convection flow over a heated horizontal cylinder, published in Applied Thermal Engineering (details can be found here). Our work has been covered in Advances in Engineering (article link), with a glimpse of the research shown below:
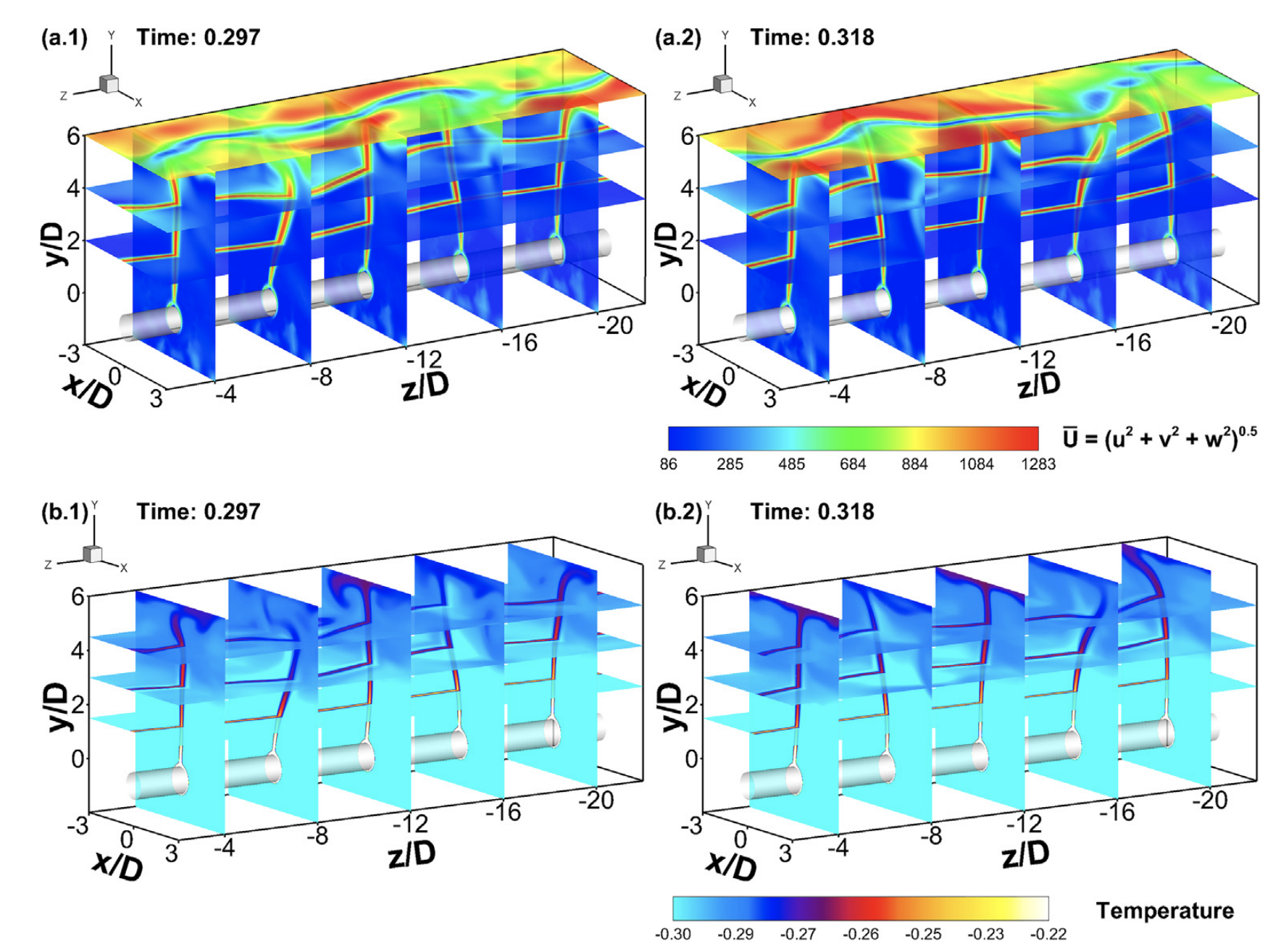
Due credits to Prof. Gazzola for developing the core of the FSI-remeshed vortex method code, which I have used to simulate some of the results shown in this website.